CRAFT’s research activities are focussed in the three following Research Pillars (research areas) which align with the National Research Council and University of Toronto’s strengths in microfluidics and offer exciting opportunities for collaborations:
Organ-on-a-Chip
Co-Leads: Milica Radisic and Teodor Veres
Aim: to deploy organ-on-chip technologies that will transform drug discovery and development and disease modelling in biomedical research.
The most commonly used model systems to study human physiological processes are cell lines and animals. However, it is becoming increasingly clear that both of these model systems fall far short in accurately predicting drug efficacy in humans, personalized drug sensitivities and off-target drug toxicity.
Recent advances in microfabrication, microfluidic devices and stem cell biology have enabled the miniaturization of 3D tissue models, more commonly known organ-on-a-chip models. Organ-chip models can better recapitulate the hallmarks of human physiology and disease in vitro. These models hold much promise and could revolutionize drug discovery and development by improving drug safety and efficacy, helping to bring personalized and more beneficial drugs to the market, and reducing the time and expenditure associated with drug development.
Example Projects:
- A tumour-on-a-chip model to examine T cell/tumour interactions (PI: Allison McGuigan)
- A lung airway-on-a-chip device to examine the effect of particular matter deposition on the mechanobiology of airflow (PI: Edmond Young)
- New imaging methods to examine the biomechanics of 3D cardiac microtissue models (PI: Craig Simmons)
CRAFT’s organ-on-chip pillar is tightly linked with the following programs based at the University of Toronto:
- Ontario-Québec Center for Organ-on-a-Chip Engineering (oqCORE), which aims to address current barriers preventing broad adoption, scalable manufacturing and commercialization of organ-chip devices
- NSERC CREATE Training Program in Organ-on-a-Chip Engineering and Entrepreneurship (TOeP), which equips trainees with knowledge and skills to build their own companies based on their organ-chip research
Biofabrication
Co-Leads: Axel Guenther and Teodor Veres
Aim: to develop biofabrication (biofab) strategies for tissue- and patient-specific structures in laboratory and preclinical settings.
Millions of people worldwide are in dire need of lifesaving treatments for damaged and/or dysfunctional organs and tissues. The World Health Organization estimates that the total number of organ transplants performed every year (~145,000) represents only 10% of the actual global need. Available treatments—such donor organs, replacement tissues and tissue engineering solutions—cannot address this gap.
Biofabrication, the automated generation of functional products consisting of living cells, biomaterials and synthetic materials, can generate these much-needed tissue and organ substitutes. However, current biofabrication technology is only capable of producing millimeter-to-centimeter-sized tissues, which is insufficient for treating damaged or dysfunctional tissues and organs in people. Most human organs are at least one order of magnitude larger in size and at least three orders of magnitude larger in volume than engineered tissues that we can currently produce in the lab. We need economical biofabrication strategies tailored to the clinic, which can produce larger amounts and bigger pieces of engineered tissues under clinical conditions and at clinically relevant rates.
Example Projects:
- 3D bioprinted retrievable stents for congenital heart disease (PI: Naomi Matsuura)
- A handheld 3D printer that can deposit sheets of engineered skin directly onto large burn wounds (PI: Axel Guenther)
- Developing a new skin substitute consisting of autologous (patient-derived) stem cells and a degradable hydrogel matrix (PI: Marc Jeschke)
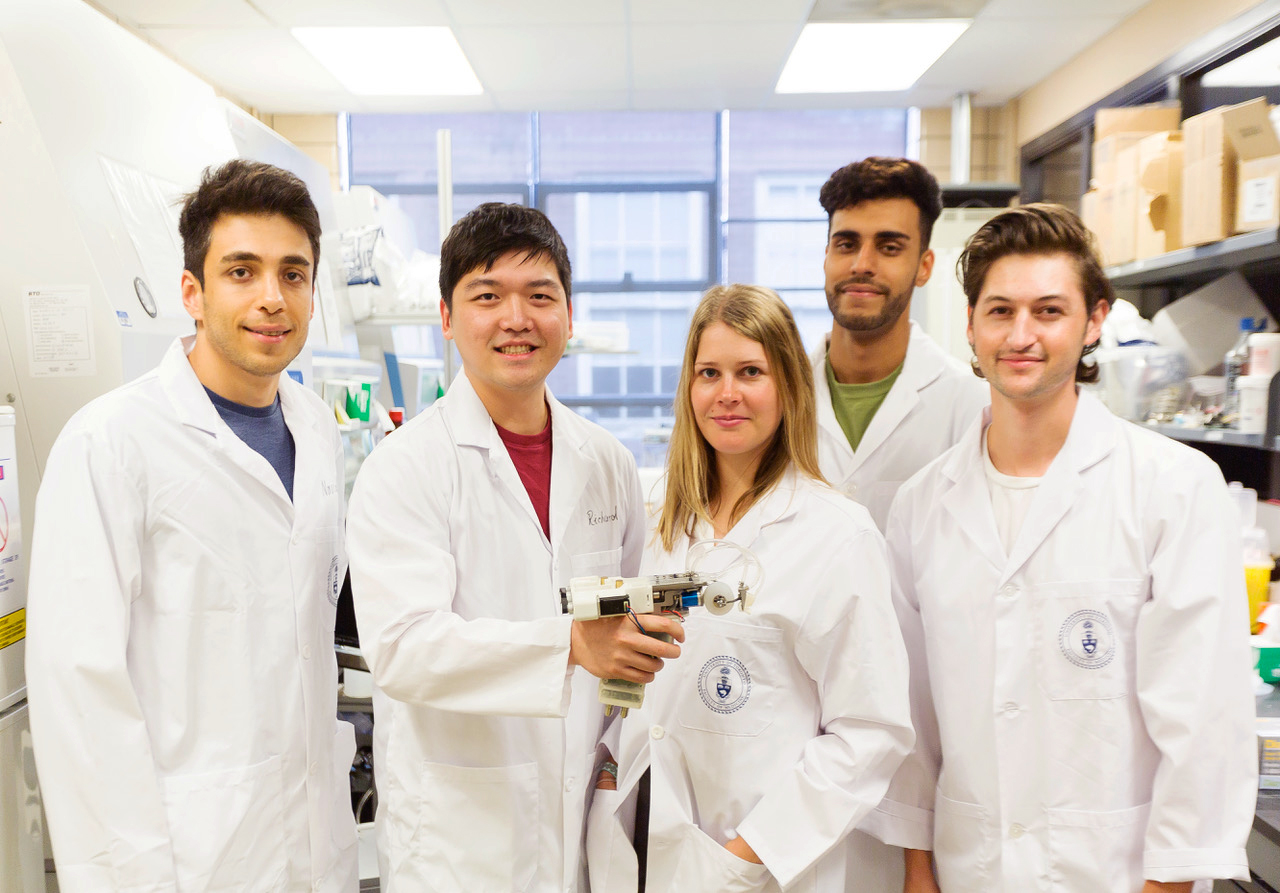
Diagnostics
Co-Leads: Aaron Wheeler and Teodor Veres
Aim: to create and deploy inexpensive diagnostic devices that will empower health care professionals to provide more timely and appropriate care anywhere, anytime and to anyone who needs it.
Most in vitro diagnostic tests—which are the cornerstone of diagnosis, prognosis and treatment—are run in centralized laboratories. They require highly trained personnel, specialized infrastructure, and expensive reagents, which mean the tests are less accessible—even inaccessible—to certain groups of people. Further, results are often slow to reach health care professionals, which delays clinical decision-making and patient management. Collectively, these factors can compromise the care of patients, putting them at higher risk of morbidity and mortality.
Microfluidic technology can significantly improve the accessibility of tests by miniaturizing and automating the laboratory procedures on a portable device (lab-on-a-chip) that can be taken directly to the patient, at point-on-care (POC), and process samples and provide results much more rapidly, efficiently, and cost effectively than using centralized laboratories.
The Diagnostics Pillar will focus on health challenges of national priority for which the use of rapid and precise diagnostics are urgently needed, including infectious diseases, antimicrobial resistance and care in remote and rural communities.
Project Examples:
- A smart phone that can detect infectious diseases (PI: Warren Chan)
- Identifying sepsis subtypes and associated biomarkers that will be detected using a bedside microfluidics-enabled diagnostic device (PI: Claudia dos Santos)
- Non-invasive prenatal diagnosis (PI: Aaron Wheeler)